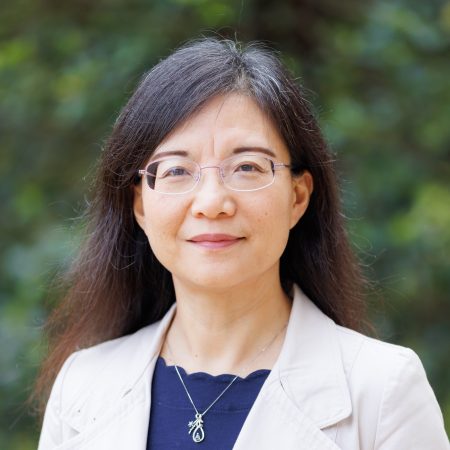
Education
- BS, Wuhan University, 1990
- PhD, Institute of Photographic Chemistry, Chinese Academy of Sciences, 1995
- Post-doctoral Associate, University of Alabama-Birmingham, 1997-1999
Research
The Sun group’s research interests lie in organometallic photonic and biophotonic materials. We have been focused on the design, synthesis and characterization of transition-metal complexes (TMCs), such as Pt(II), Ir(III), and Cu(I) complexes, for optical limiting, photodynamic therapy (PDT), optical sensing, organic light emitting devices (OLEDs), and bioimaging applications. We aim to understand the structure-property correlations in these complexes for rational design of new complexes with predetermined properties. My research program is highly interdisciplinary, embracing organic synthesis, coordination chemistry, spectroscopic studies, theoretical simulations, nonlinear optical characterizations, and photosensitization mechanistic studies. The current major research projects include:
- Developing NIR-absorbing theranostic Ir(III) complexes for imaging-guided photodynamic therapy and photothermal therapy
Photodynamic therapy (PDT) and photothermal therapy (PTT) are emerging cancer treatment modalities based on the photosensitizers (PSs) to convert photon energy into reactive oxygen species (ROS) or heat. PDT/PTT treatment only becomes effective when the PS is activated by light and in the presence of oxygen in case of PDT. Therefore, one can irradiate only at the diseased tissue to avoid damage to the normal tissues. Unfortunately, the widespread use of PDT for cancer treatment has been limited by the drawbacks of the only FDA-approved PS for cancer PDT, i.e. Photofrin®, such as long cutaneous photosensitivity, weak absorption at its clinical wavelength (630 nm) and virtually no absorption of tissue-penetrating NIR light, and its ineffectiveness in hypoxia due to its mechanistic reliance on O2. Development of new PSs that can specifically target tumors, absorb strongly in the NIR region (700-850 nm), and have very long-lived triplet excited states for efficient ROS production in hypoxia are urgently needed, especially for treating deep seated and/or solid tumors. Certain heavy TMCs, such as those based on Ir(III), Ru(II), Os(II), and Pt(II), have attracted great attention in recent years because of their (1) rich photophysical properties, (2) tunable excited state dynamics, (3) high quantum yields for triplet state formation due to metal-induced rapid intersystem crossing, and (4) better stabilities leading to decreased dark toxicities.
To optimize the PDT/PTT treatment, it is better to apply light irradiation when the PS reaches maximum accumulation at the tumor site, which can be tracked by bioimaging. NIR emitting PSs are desirable bioimaging materials because the NIR emission has deeper tissues penetration, less photodamage to biological samples, and reduced interference from cell auto-fluorescence and tissue absorption. TMCs like Ir(III) complexes exhibit improved NIR emission quantum yield, feasible tunability of the emission color, and good photostability and permeability through cell membranes. Thus, they are excellent candidates for bioimaging applications as well.
We have designed and investigated a variety of mononuclear and dinuclear Ir(III) complexes with trisbidentate or bisterdentate ligands for theranostic PDT/PTT applications, and systematically explored the structure-photophysical property-biological activity correlations. Recently, a novel neutral Ir(III) complex absorbing at 670 nm was developed in our group and encapsulated in polymeric micelles. The micelles show a high in vivo tumor accumulation and low dark toxicity, and completely destroy the orthotopic 4T1-Luc tumors on mice and remarkably prevent lung metastasis from the primary tumors via synergetic PDT/PTT effects upon 660 nm light irradiation. These results demonstrate the promising of applying Ir(III) PS for effective treatment of hypoxic solid tumors by supplementing the oxygen-dependent PDT process with oxygen-independent PTT in hypoxia. To further red-shift the absorption to the NIR region and increase the triplet excited state lifetime of Ir(III) PSs, as well as to enhance their cancer selectivity, we are currently developing novel Ir(III) PSs incorporating chalcogenophene-substituted diketopyrrolopyrrole motif and folic acid for treating melanoma and triple negative breast cancers via a combination of PDT and PTT. Meanwhile, we plan to increase the local oxygen tension in solid tumors via encapsulation of our NIR-absorbing Ir(III) PS into cancer specific targeting, H2O2-activatable, and O2-evolving micelles to efficiently produce ROS in hypoxia and thus further enhance the PDT/PTT efficiency in hypoxic tumors. Our long-term objective is to advance the PDT/PTT modality as a more effective and much safer cancer therapy for hard-to-cure tumors.
- Data-driven and computationally assisted design of near-infrared emissive metal-organic complexes with earth-abundant metals
In addition to the aforementioned applications in PDT and bioimaging, Ir(III) complexes have been widely studied for OLEDs. However, Ir is a precious metal with very low natural abundance, which significantly increases the cost of devices based on the Ir(III) complexes. Meanwhile, development of efficient triplet NIR-emitting TMCs that have potential applications in night-vision-readable displays, telecommunication, optical sensing, and bioimaging etc. has been under-pursued to date. This is due to the limited understanding of interplays between the electronic excitation that promotes radiative passways and those responsible for molecular vibrations that govern the non-radiative processes.
This project aims to develop efficient NIR emitting TMCs based on earth abundant transition metals copper (Cu), tungsten (W), and molybdenum (Mo). To realize this goal, the relationships between the geometrical structures and emission characteristics need to be fully understood, which is a great challenge for conventional experimental approaches because numerous ligand combinations should be studied. Instead of tedious experimental studies to select the best ligand combinations, a methodology that combines experimental data and computational results with data-driven analysis based on machine learning (ML) and cheminformatic models will greatly speed up the selection process. This approach will elucidate key structural factors responsible for NIR emission and the predicted criteria will be used to guide the rational design of novel NIR-emitting TMCs with Cu, Mo, and W metals. This collaborative project involves experimentalists (my group), computational chemists and cheminformatic researchers. My group will be in charge of the design, synthesis, and photophysical studies of several series of Cu(I), Mo(0), and W(0) complexes bearing diimine ligands with different substituents and varied degrees of p-conjugation. Such a detailed characterization of the photophysics of TMCs is critical for advancing our understanding of the structure-property correlations and thus promotes our ability in designing new NIR-emitting TMCs.
- Developing AIE (aggregation-induced emission)-active, multistimuli-responsive Pt(II) and Ir(III) complexes for bioimaging, sensing or OLED applications
AIE is a unique phenomenon that the emission intensity of a compound is enhanced in its aggregated form compared to that in solution due to restricted motion (RM) in aggregates, which has potential applications in bioimaging and OLEDs. For TMCs with appropriate functional groups on the ligand(s) enabling RM in aggregates, the increased intermolecular interactions (p–p or metal-metal interactions) can change the energy and/or nature of the lowest emitting excited state and induce blue- or red-shift of the emission in aggregates. Thus, the origins of the AIE effects in TMCs are more complicated and under-explored. A better understand of the AIE effects in TMCs is needed for rational design of new complexes with enhanced AIE effects. Mechanochromism, vapochromism, and thermochromism are phenomena showing distinct color or emission color/intensity/lifetime change upon mechanical, organic vapor, or temperature stimulations. These phenomena have potential applications in pressure, vapor, or thermal sensors, anticounterfeit, food safety and security, and biosystem monitoring. These effects, along with the AIE effect are all closely related to the molecular packing and conformation. However, how to control the molecular packing or conformation in aggregates/solid state to obtain desired properties remains to be a great challenge, especially in TMCs that have not been well studies.
In this project, we aim to build up the structure-property correlations in Pt(II) and Ir(III) complexes for rational design of these TMCs with predetermined AIE, mechanochromic, vapochromic, or thermochromic effects. Pt(II) complexes are selected for this study because of their square-planar configuration that facilitates the intermolecular interactions in aggregates/ solid state via ligand p–p or metal-metal interactions. While the octahedral configuration of Ir(III) complexes prevents intermolecular metal-metal interactions, intermolecular ligand p–p interactions are feasible. We have demonstrated that some Ir(III) complexes with “loose” packing exhibited distinct mechanochromic/vapochromic effects upon grinding or vapor fuming. We also discovered that the molecular packing and intermolecular interactions are impacted by the alkyl chain length and branching on the bipyridine ligand in Pt(II) complexes. We anticipate the success of this project will provide a better understanding on controlling the molecular packing and conformation in Pt(II) and Ir(III) complexes and the structure-property correlations discovered could be applied to other square-planar or octahedral TMCs.
- Developing heavy transition-metal complexes for broadband optical limiting applications
Nonlinear absorbing materials with broad and strong reverse saturable absorption (RSA, a nonlinear absorption process in which a molecule absorbs more photons in its excited state than in its ground state) are desired for various photonic applications such as optical limiting, optical switching, laser mode locking, optical pulse shaping and spatial light modulation, especially for protection of human eyes and optical sensors from laser damages. The effectiveness of RSA is mainly governed by ratios of the absorption cross sections between the excited and ground states. Efficient RSA of nanosecond laser pulses also requires a long-lived triplet excited state (T1) and high T1 quantum yield. For practical applications, broadband RSA materials are required to cover the spectral range of 400-900 nm and be effective toward a range of pulsed and continuous lasers. Heavy TMCs, such as Pt(II) and Ir(III) complexes are attractive for this purpose because the strong spin-orbit coupling constants for the d orbitals of Pt(II) and Ir(III) can lead to high T1quantum yields not only through rapid intersystem crossing but also through direct transitions from the singlet ground state (S0) to the T1 state in the case of Ir(III) complexes. The spin-forbidden S0®T1 absorption in Ir(III) complexes can also induce broad and weak ground-state absorption in the 450-850 nm region when appropriate ligands are used, which is a desirable feature for broadband reverse saturable absorbers. Many of the Pt(II) and Ir(III) complexes possess broadband excited-state absorption in the visible to the NIR regions; and the structures of these complexes can be readily modified via selection of appropriate ligands. We have systematically investigated the structure-property correlations in a variety of Pt(II) and Ir(III) complexes bearing terdentate or bidentate ligands and are especially interested in understanding the impacts of ligand p-conjugation on the ground- and excited-state absorption and on the triplet excited-state lifetime, aiming to obtain broadband reverse saturable absorbers for optical limiting applications.
Selected Publications
Dr. Sun’s full publication list